Review Article | Open Access
Mid-life: a critical window for intervention during aging
Evandro Lopes de Carvalho1
1Agostinho Neto University Hospital, Praia, Cape Verde.
Correspondence: Evandro Lopes de Carvalho (Agostinho Neto University Hospital, Praia, Cape Verde; E-mail: evandro.l.carvalho@huan.gov.cv).
Asia-Pacific Journal of Surgical & Experimental Pathology 2025, 2: 1-8. https://doi.org/10.32948/ajsep.2025.01.08
Received: 23 Nov 2024 | Accepted: 07 Jan 2025 | Published online: 14 Jan 2025
Key words aging, mid-life, plasma proteome dynamics, adipose remodeling, aging biomarkers
Lehallier et al. [5] also observed that not all proteins follow a simple linear trajectory, but many exhibit nonlinear patterns, including exponential rises and/or stepwise increases. For instance, proteins associated with axon guidance pathway [10] and EPH-ephrin signaling [11, 12] show steady levels until mid-life before undergoing exponential changes. When metabolic regulation becomes critically imbalanced, particularly. These findings of non-linear proteomic changes challenge the traditional models of aging that often assume a gradual, uniform decline. The coordinated but heterogeneous nature of aging is corroborated with clustering of proteins into distinct trajectories, ranging from linear patterns to exponential and logarithmic changes. For instance, proteins involved in blood microparticles showed consistent decline with age (as further explored by Allan et al. [13]), while proteins linked to glycosaminoglycan binding and heparin activity increased in a stepwise manner during aging. These varying patterns suggest that age-related metabolic stressors activate or suppress specific biological pathways, particularly during mid-life [5].
Lehallier et al. [5] further enriched our understanding regarding gender-specific differences in proteomic aging. Distinct proteomic profiles were present in male and female participants, heavily influenced by hormonal variations during aging. For instance, proteins associated with follicle-stimulating hormone [14] and prostate-specific antigen [15] exhibited significant sex-specific differences. In line with these findings, increase in follicle-stimulating hormone levels is now established as key determining factor of accelerated ovarian aging [14]. Such variations are also associated with different metabolic and physiological pathways in a sex-specific manner during aging. A key example here is the sudden hormonal shifts observed during menopause are experienced by women, worsening their metabolic vulnerabilities [16], whereas a more gradual decline in androgens affects metabolic health in men [17]. Hence, sex-specific proteomic markers identified may aid in devising tailored interventions targeting these distinct hormonal and metabolic trajectories.
Lehallier et al. [5] also identified aging and metabolic dysregulation-related specific plasma proteins, such as growth differentiation factor 15 (GDF15) and sclerostin. Increased GDF15 expression with age is linked to metabolic stress responses and all-cause mortality. Based on its association with conditions like obesity and type 2 diabetes, this increase in GDF15 levels may serve as a valuable biomarker for detecting early signs of metabolic dysregulation during mid-life [18, 19]. On the other hand, sclerostin is a protein related to bone metabolism and systemic metabolic health [20, 21]. These proteins may serve as indicators of metabolic stress, offering potential therapeutic targets for mitigating mid-life transition. Lehallier et al. [5] further highlighting conserved aging-related proteins that exhibit similar trajectories in humans and mice by strengthening their findings through incorporating data from mouse models. Notably, older mice that were given plasma from younger mice had a rejuvenated proteomic profile, whereas younger mice receiving plasma from older mice had signs of accelerated aging. This cross-species validated phenomenon highlights the evolutionary roots of metabolic aging and confirms the proteomic alterations seen in humans.
Biological age represents the true physiological condition of an individual in contrast to chronological age that simply measures the passage of time [22]. A predictive proteomic clock developed by Lehallier et al. [5], comprising 373 proteins, estimated the biological age with high accuracy. This proteomic clock has revealed that biologically young individuals performed better on physical and cognitive tests compared to their chronologically aged controls, suggesting a link between proteomic health and overall functional status. In future, this clock can serve as a tool to evaluate metabolic health and development of age-related diseases in mid-life individuals. Lehallier et al. [5] also Introduced an analytical tool called differential expression-sliding window analysis (DE-SWAN) that offered solution to dissect the proteomic changes at a high resolution. By revealing hundreds of proteins that fluctuate in distinct waves over the course of life, this method detected stage-specific variations in protein expression, highlighting the intricate nature of the aging process. For example, significant age-related changes in chordin-like protein 1 (CHRDL1) and matrix metallopeptidase 12 (MMP12) during later stages of life reflect tissue remodeling and metabolic decline. Of note, MMP12 has been identified as a key determinant of mechanical homeostasis in the aorta during aging [23].
Overall, Lehallier et al. [5] revealed the fluctuating and nonlinear patterns of proteomic changes during aging, establishing plasma proteome as key determinant of aging process and offering a deeper understanding of the molecular foundations of the mid-life transition (Figure 1 and Table 1). Identifying crucial proteins and pathways influenced during mid-life, Lehallier et al. [5] has opened up important possibilities for creating targeted treatments and personalized strategies. Cross-species validation and development of the proteomic clock highlight the potential of using plasma proteome profiling as a diagnostic and therapeutic tool, opening the door to innovative strategies for enhancing healthspan and tackling the challenges associated with aging.
Table 1. Biomarkers of mid-life transition. |
||||
Category |
Biomarker |
Role |
Evidence/Mechanism |
Ref |
Plasma proteome |
GDF15 |
Marker of metabolic stress (linked to obesity and type 2 diabetes) and all-cause mortality |
Increased expression with aging associated with mid-life metabolic dysregulation |
[5] |
Sclerostin |
Involved in bone metabolism and systemic metabolic health |
Elevated levels, particularly during mid-life, impact metabolic processes |
||
CHRDL1 |
Reflects tissue remodeling and metabolic decline |
Nonlinear expression pattern with significantly upregulated during mid-life which is persistent onwards |
||
MMP12 |
Linked to aortic mechanical homeostasis and aging-related metabolic changes |
Nonlinear expression pattern with significant upregulation during mid-life which is persistent onwards |
||
Immune system |
Naïve T Cells |
Decline correlates with adaptive immune system weakening and inflammaging |
Sex-specific immune aging where men show sharper declines compared to women |
[6] |
IL18 |
Pro-inflammatory marker contributing to metabolic syndrome and cardiovascular diseases |
Higher expression in men during mid-life, leading to inflammatory stress |
||
IL1B |
Pro-inflammatory marker linked to insulin resistance and chronic inflammation |
Elevated expression exacerbates metabolic dysfunction during mid-life |
||
Granzyme B |
Cytotoxic molecule indicating increased inflammatory state in aging |
Reflects enhanced immune activation and inflammaging during mid-life |
||
Adipose tissue remodeling |
CCL8 |
Pro-inflammatory cytokine involved in immune cell infiltration and inflammation in adipose tissue |
Upregulated during mid-life, triggering systemic inflammation |
[7] |
VCAM1 |
Marker of immune cell adhesion and tissue inflammation |
Elevated levels during mid-life indicate adipose-driven systemic inflammation |
||
Periostin |
Regulates extracellular matrix and lipid metabolism |
Decreased levels during mid-life are linked to systemic metabolic effects |
||
Systemic cellular senescence |
β-galactosidase |
Marker of senescent cells and systemic aging |
Elevated levels during mid-life correlates with cellular stress and inflammation |
[8] |
p16 and p21 |
Markers of senescence and age-related metabolic stress |
Increased expression contributes to tissue remodeling and dysfunction |
||
*Glb1-2A-mCherry (GAC) |
Signal for systemic aging, predicting lifespan and organ-specific aging |
Elevated in mid-life and is linked to cardiac hypertrophy and metabolic stress |
||
* Glb1-2A-mCherry (GAC) is a synthetic marker established to track aging in vivo. |
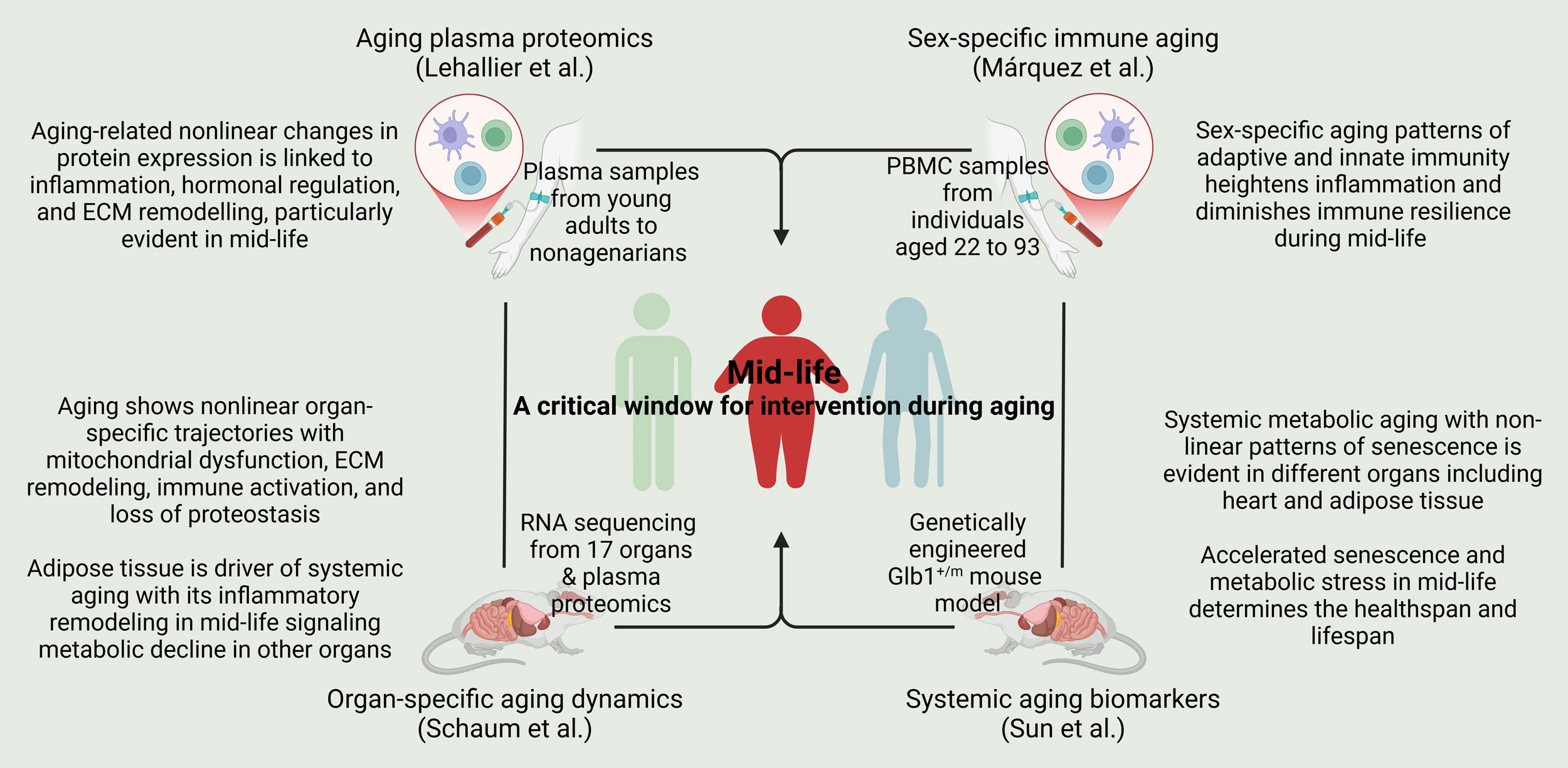
Sex-specific nature of immune system aging is a key finding by Márquez et al. [6], which is in line with those by Lehallier et al. [5] who observed gender-specific differences in proteomic aging. Women tend to have stronger adaptive immune responses (higher levels of naïve T cells and B cells), along with better thymic function throughout their lives. On the other hand, men experience a sharper drop in adaptive immunity and a noticeable rise in innate immune activity (particularly in monocytes and pro-inflammatory cytokines) [6, 25]. These differences are most pronounced after the age of 65, where men exhibit enhanced innate immune responses while women maintain relatively robust adaptive immune function [6]. These findings provide a framework to understand why men and women experience different susceptibilities to metabolic and inflammatory conditions during mid-life and beyond. Márquez et al. [6] also explored the molecular underpinnings of these changes, identifying specific genomic regions and pathways that are differentially affected by aging in men and women. In men, there is a significant loss of chromatin accessibility and gene expression in B-cell-specific loci, correlating with reduced B-cell proportions in PBMCs [26]. In contrast, women show only a slight activation of B-cell-related genes and loci, allowing them to sustain higher B-cell levels even as they age. These differences between sexes in B cells help explain why women are more prone to autoimmune conditions. Meanwhile, the age-related decline in humoral immunity tends to be more pronounced in men [27]. Another notable aspect of the study by Márquez et al. [6] is the identification of conserved aging signatures across sexes, characterized by the loss of naïve T-cell function and the activation of cytotoxic cells, including natural killer (NK) cells and memory CD8+ T cells [28]. These changes reflect the immune system shifts from adaptive to innate immunity as we age, a key feature of immunosenescence. The study points to important molecules like [29] and CCR7 [30], which become less active over time and weaken T-cell function. On top of that, the activation of cytotoxic pathways involving granzyme B and perforin highlights the growing pro-inflammatory state that comes with aging [31, 32]. This inflammation, often called "inflammaging", can have ripple effects on metabolism and overall health.
Márquez et al. [6] employed advanced computational approaches to delineate temporal patterns of immune aging. The study looked at changes in chromatin accessibility and gene expression over time and found three distinct patterns. Each pattern is tied to specific cell types and their roles in the body. These clusters reveal that the loss of adaptive immune features and the gain of innate immune characteristics occur in a phased manner, with abrupt shifts during the identified breakpoints in the late 30s and mid-60s. The timing of these changes is similar for both sexes, but the intensity and the cell types involved differ, highlighting the role of sex in immune aging. The mid-life decrease in adaptive immunity and increase in inflammation may contribute to a systemic environment, disrupting overall metabolism [33]. Increased expression of pro-inflammatory cytokines, such as IL18 [34, 35] and IL1B [36, 37], and their receptors in men suggest their greater susceptibility to chronic inflammatory conditions, driving metabolic syndrome, insulin resistance, and cardiovascular disease. Women have stronger adaptive immunity, which offers some protection to them, but hormonal changes during menopause increase their risk of metabolic conditions, showing sex-specific interplay between hormonal shifts and immune aging [6].
Overall, Márquez et al. [6] provided a comprehensive analysis of immune aging and their implications for mid-life transition, shedding light on molecular and cellular changes behind immune system shifts during aging, and offering new insights into how aging, immunity, and metabolism are interconnected (Figure 1 and Table 1). In addition, the sex-dimorphic nature of these changes highlights the importance of tailored interventions [38] that account for the unique aging trajectories of men and women, offering a roadmap for future research and clinical applications.
Schaum et al. [7] identified adipose tissue as key culprit in driving systemic aging. Adipose tissue as a critical site where immune cell infiltration begins early in the aging process, preceding similar changes in other organs [48, 49]. This early infiltration is marked by the activation of cytokine-mediated inflammatory pathways, with genes such as CCL8 showing significant upregulation [50]. The research showed that body fat plays a major role in storing inflammation throughout the body, which is a key factor in the metabolic issues often seen in mid-life. Fat stores, particularly around the gonads and under the skin, experience significant changes at both the cellular and genetic levels during this period, such as increase in immune cells like T cells and B cells, as well as the activation of pathways that trigger inflammation [51]. Single-cell RNA sequencing identified that immunoglobulin J (IgJ) expressing plasma B increase in number with age, especially in tissues like visceral fat. Here, higher levels of inflammatory factors and onset of unfolded protein response, a sign of endoplasmic reticulum stress, confer state of chronic inflammation [52-54] in the adipose tissue during mid-life.
The systemic impact of adipose tissue remodeling was evident from correlations between plasma protein levels and organ-specific gene expression in Schaum et al.’s findings [7]. Plasma proteins like vascular cell adhesion molecule 1 (VCAM1) and periostin are associated with differential gene expression in adipose tissue and other organ, suggesting their role in communication between different organs during aging [55, 56]. VCAM1 is particularly implicated in immune cell adhesion and tissue inflammation [57], whereas periostin plays a role in extracellular matrix regulation and lipid metabolism [56]. Hence, adipose tissue play important role in systemic aging throughout the body, with it molecular signals affecting distant organs and contributing to overall metabolic decline [7]. Schaum et al. [7] also observed circadian rhythm disruption [58] as genes controlling body's internal clock, such as Arntl [59, 60] and Npas2 [61, 62], were found to be dysregulated in various tissues during aging process, which can lead to metabolic dysfunction and inflammation. These disturbances were particularly noticeable in fat tissue, leading to dysregulated interaction with immune system and poor extracellular matrix maintenance [63], highlighting that disruptions in fundamental biological rhythms during mid-life exacerbate systemic aging processes.
Overall, Schaum et al. [7] offered a comprehensive view of the systemic and organ-specific dynamics of aging, shedding light on the molecular and cellular processes that underlie mid-life transition, emphasizing the key role of adipose tissue in driving systemic inflammation and metabolic imbalances (Figure 1 and Table 1). Mid-life represented a critical juncture as well where multiple aging pathways converged, amplifying metabolic stress and vulnerability. This eventually highlighted the importance of tailored interventions (such as those towards adipose tissue) to tackle the complex factors influencing aging.
Cellular senescence is associated with elevated levels of β-galactosidase, p16, and p21, contributing to chronic inflammation and tissue remodeling during aging [68, 69]. A rise in GAC signal intensity as observed by Sun et al. [8] was associated with increased levels of β-galactosidase, reflecting increased cellular senescence. As cardiomyocytes lose their proliferative capacity in adulthood, they adapt to stress by undergoing hypertrophic growth rather than division. This response, while initially compensatory, becomes pathological over time, contributing to heart failure and metabolic dysregulation [70]. GAC signal efficiently predicted cardiac hypertrophy as a key feature of mid-life transition. Sun et al. [8] demonstrated that mice with higher GAC signals during middle age exhibited thicker left ventricular walls and reduced cardiac output, signaling early stages of heart dysfunction. These changes, occurring independently of chronological age, highlight the role of biological aging as a driver of mid-life transition.
The GAC signal as observed by Sun et al. [8] was detectable in multiple tissues, including the brain, liver, kidney, and spleen, highlighting the widespread impact of aging on metabolic and physiological systems and underscoring the systemic nature of aging. Moreover, accumulation of senescent cells in key metabolic organs such as the heart and adipose tissue created a pro-inflammatory microenvironment that exacerbated metabolic stress during mid-life [71, 72]. The further highlighted the interconnected nature of mid-life transition, where dysfunction in one organ system can impact distant organs as well. For instance, senescent cells in adipose tissue secrete pro-inflammatory cytokines that exacerbate insulin resistance and cardiovascular dysfunction, creating a vicious cycle of metabolic decline [72, 73]. Sun et al. [8] also observed nonlinear nature of aging, with the GAC signal displaying a sharp rise during middle age before plateauing or declining in late age. Thus, middle age represents a distinct phase in the aging trajectory, characterized by accelerated senescence and metabolic stress. Interventions targeting aging in mid-life could yield significant benefits in delaying the onset of age-related diseases and extending healthspan [74]. In particular, Sun et al. [8] found that senolytic treatments with dasatinib and quercetin (D+Q) [75] effectively reduced the GAC signal in pathological senescence models, such as bleomycin-induced lung injury, highlighting the therapeutic potential of targeting senescent cells to alleviate the metabolic challenges of mid-life. Moreover, the use of the GAC signal as a live-imaging marker allowed for real-time monitoring of aging processes, providing a valuable tool for evaluating the efficacy of anti-aging therapies [8].
Overall, Sun et al. [8] provided a comprehensive framework for understanding the systemic nature of aging and its impact on mid-life metabolic health. By establishing the GAC signal as a dynamic marker of aging, the research highlighted the importance of targeting senescent cells and mitigating systemic aging during middle age (Figure 1 and Table 1). Identification of individuals with high aging signals during mid-life can benefit clinicians to device and implement targeted therapies and mitigate metabolic and cardiovascular risks, extending healthspan and reducing the burden of age-related diseases.
No applicable.
Ethics approval
No applicable.
Data availability
The data will be available upon request.
Funding
None.
Authors’ contribution
ELC contributed to the conception, design, writing of this review article, drawing figures, make data table and submitted the final version of the manuscript.
Competing interests
None.
- López-Otín C, Blasco MA, Partridge L, Serrano M, Kroemer G: Hallmarks of aging: An expanding universe. Cell 2023, 186(2): 243-278.
- Guo J, Huang X, Dou L, Yan M, Shen T, Tang W, Li J: Aging and aging-related diseases: from molecular mechanisms to interventions and treatments. Signal Transduct Target Ther 2022, 7(1): 391.
- Lachman ME, Teshale S, Agrigoroaei S: Midlife as a Pivotal Period in the Life Course: Balancing Growth and Decline at the Crossroads of Youth and Old Age. Int J Behav Dev 2015, 39(1): 20-31.
- Timmons JA, Volmar CH, Crossland H, Phillips BE, Sood S, Janczura KJ, Törmäkangas T, Kujala UM, Kraus WE, Atherton PJ, et al: Longevity-related molecular pathways are subject to midlife "switch" in humans. Aging Cell 2019, 18(4): e12970.
- Lehallier B, Gate D, Schaum N, Nanasi T, Lee SE, Yousef H, Moran Losada P, Berdnik D, Keller A, Verghese J, et al: Undulating changes in human plasma proteome profiles across the lifespan. Nat Med 2019, 25(12): 1843-1850.
- Márquez EJ, Chung CH, Marches R, Rossi RJ, Nehar-Belaid D, Eroglu A, Mellert DJ, Kuchel GA, Banchereau J, Ucar D: Sexual-dimorphism in human immune system aging. Nat Commun 2020, 11(1): 751.
- Schaum N, Lehallier B, Hahn O, Pálovics R, Hosseinzadeh S, Lee SE, Sit R, Lee DP, Losada PM, Zardeneta ME, et al: Ageing hallmarks exhibit organ-specific temporal signatures. Nature 2020, 583(7817): 596-602.
- Sun J, Wang M, Zhong Y, Ma X, Sun S, Xu C, Peng L, Li G, Zhang L, Liu Z, et al: A Glb1-2A-mCherry reporter monitors systemic aging and predicts lifespan in middle-aged mice. Nat Commun 2022, 13(1): 7028.
- Sun BB, Chiou J, Traylor M, Benner C, Hsu YH, Richardson TG, Surendran P, Mahajan A, Robins C, Vasquez-Grinnell SG, et al: Plasma proteomic associations with genetics and health in the UK Biobank. Nature 2023, 622(7982): 329-338.
- van Erp S, van Berkel AA, Feenstra EM, Sahoo PK, Wagstaff LJ, Twiss JL, Fawcett JW, Eva R, Ffrench-Constant C: Age-related loss of axonal regeneration is reflected by the level of local translation. Exp Neurol 2021, 339: 113594.
- Zheng S, Sun F, Tian X, Zhu Z, Wang Y, Zheng W, Liu T, Wang W: Roles of Eph/ephrin signaling pathway in repair and regeneration for ischemic cerebrovascular and cardiovascular diseases. J Neurorestoratol 2023, 11(1): 100040.
- Popov C, Kohler J, Docheva D: Activation of EphA4 and EphB2 Reverse Signaling Restores the Age-Associated Reduction of Self-Renewal, Migration, and Actin Turnover in Human Tendon Stem/Progenitor Cells. Front Aging Neurosci 2015, 7: 246.
- Allan HE, Hayman MA, Marcone S, Chan MV, Edin ML, Maffucci T, Joshi A, Menke L, Crescente M, Mayr M et al: Proteome and functional decline as platelets age in the circulation. J Thromb Haemost 2021, 19(12): 3095-3112.
- Mehalko K, Kim M, Paye S, Koh K, Lu RJ, Benayoun BA: Lack of accelerated ovarian aging in a follicle-stimulating hormone receptor haploinsufficiency model. Transl Med Aging 2023, 7: 1-8.
- Matti B, Xia W, van der Werf B, Zargar-Shoshtari K: Age-Adjusted Reference Values for Prostate Specific Antigen - A Systematic Review and Meta-Analysis. Clin Genitourin Cancer 2022, 20(2): e114-e125.
- Motlani V, Motlani G, Pamnani S, Sahu A, Acharya N: Endocrine Changes in Postmenopausal Women: A Comprehensive View. Cureus 2023, 15(12): e51287.
- Barrientos G, Llanos P, Basualto-Alarcón C, Estrada M: Androgen-Regulated Cardiac Metabolism in Aging Men. Front Endocrinol (Lausanne) 2020, 11: 316.
- Wiklund FE, Bennet AM, Magnusson PK, Eriksson UK, Lindmark F, Wu L, Yaghoutyfam N, Marquis CP, Stattin P, Pedersen NL et al: Macrophage inhibitory cytokine-1 (MIC-1/GDF15): a new marker of all-cause mortality. Aging Cell 2010, 9(6): 1057-1064.
- Asrih M, Wei S, Nguyen TT, Yi HS, Ryu D, Gariani K: Overview of growth differentiation factor 15 in metabolic syndrome. J Cell Mol Med 2023, 27(9): 1157-1167.
- Xu Y, Gao C, He J, Gu W, Yi C, Chen B, Wang Q, Tang F, Xu J, Yue H et al: Sclerostin and Its Associations With Bone Metabolism Markers and Sex Hormones in Healthy Community-Dwelling Elderly Individuals and Adolescents. Front Cell Dev Biol 2020, 8: 57.
- Dreyer TJ, Keen JA, Wells LM, Roberts SJ: Novel insights on the effect of sclerostin on bone and other organs. J Endocrinol 2023, 257(2): e220209.
- Bafei SEC, Shen C: Biomarkers selection and mathematical modeling in biological age estimation. NPJ Aging 2023, 9(1): 13.
- Spronck B, Ramachandra AB, Moriyama L, Toczek J, Han J, Sadeghi MM, Humphrey JD: Deletion of matrix metalloproteinase-12 compromises mechanical homeostasis and leads to an aged aortic phenotype in young mice. J Biomech 2022, 141: 111179.
- Baechle JJ, Chen N, Makhijani P, Winer S, Furman D, Winer DA: Chronic inflammation and the hallmarks of aging. Mol Metab 2023, 74: 101755.
- Calabrò A, Accardi G, Aiello A, Caruso C, Candore G: Sex and gender affect immune aging. Front Aging 2023, 4: 1272118.
- Moroney JB, Vasudev A, Pertsemlidis A, Zan H, Casali P: Integrative transcriptome and chromatin landscape analysis reveals distinct epigenetic regulations in human memory B cells. Nat Commun 2020, 11(1): 5435.
- Fink AL, Klein SL: The evolution of greater humoral immunity in females than males: implications for vaccine efficacy. Curr Opin Physiol 2018, 6: 16-20.
- Quinn KM, Vicencio DM, La Gruta NL: The paradox of aging: Aging-related shifts in T cell function and metabolism. Semin Immunol 2023, 70: 101834.
- Kim HR, Hong MS, Dan JM, Kang I: Altered IL-7Ralpha expression with aging and the potential implications of IL-7 therapy on CD8+ T-cell immune responses. Blood 2006, 107(7): 2855-2862.
- Da Mesquita S, Herz J, Wall M, Dykstra T, de Lima KA, Norris GT, Dabhi N, Kennedy T, Baker W, Kipnis J: Aging-associated deficit in CCR7 is linked to worsened glymphatic function, cognition, neuroinflammation, and β-amyloid pathology. Sci Adv 2021, 7(21): eabe4601.
- Richardson KC, Jung K, Matsubara JA, Choy JC, Granville DJ: Granzyme B in aging and age-related pathologies. Trends Mol Med 2024, 30(12): 1165-1179.
- Zöphel D, Angenendt A, Kaschek L, Ravichandran K, Hof C, Janku S, Hoth M, Lis A: Faster cytotoxicity with age: Increased perforin and granzyme levels in cytotoxic CD8(+) T cells boost cancer cell elimination. Aging Cell 2022, 21(8): e13668.
- Yu W, Yu Y, Sun S, Lu C, Zhai J, Lei Y, Bai F, Wang R, Chen J: Immune Alterations with Aging: Mechanisms and Intervention Strategies. Nutrients 2024, 16(22): 3830.
- Jefferis BJ, Papacosta O, Owen CG, Wannamethee SG, Humphries SE, Woodward M, Lennon LT, Thomson A, Welsh P, Rumley A, et al: Interleukin 18 and coronary heart disease: prospective study and systematic review. Atherosclerosis 2011, 217(1): 227-233.
- Trøseid M, Seljeflot I, Arnesen H: The role of interleukin-18 in the metabolic syndrome. Cardiovasc Diabetol 2010, 9: 11.
- Lagathu C, Yvan-Charvet L, Bastard JP, Maachi M, Quignard-Boulangé A, Capeau J, Caron M: Long-term treatment with interleukin-1beta induces insulin resistance in murine and human adipocytes. Diabetologia 2006, 49(9): 2162-2173.
- Szekely Y, Arbel Y: A Review of Interleukin-1 in Heart Disease: Where Do We Stand Today? Cardiol Ther 2018, 7(1): 25-44.
- Gao H, Nepovimova E, Adam V, Heger Z, Valko M, Wu Q, Kuca K: Age-associated changes in innate and adaptive immunity: role of the gut microbiota. Front Immunol 2024, 15: 1421062.
- Li Y, Berliocchi L, Li Z, Rasmussen LJ: Interactions between mitochondrial dysfunction and other hallmarks of aging: Paving a path toward interventions that promote healthy old age. Aging Cell 2024, 23(1): e13942.
- Statzer C, Park JYC, Ewald CY: Extracellular Matrix Dynamics as an Emerging yet Understudied Hallmark of Aging and Longevity. Aging Dis 2023, 14(3): 670-693.
- Cisneros B, García-Aguirre I, Unzueta J, Arrieta-Cruz I, González-Morales O, Domínguez-Larrieta JM, Tamez-González A, Leyva-Gómez G, Magaña JJ: Immune system modulation in aging: Molecular mechanisms and therapeutic targets. Front Immunol 2022, 13: 1059173.
- Meller A, Shalgi R: The aging proteostasis decline: From nematode to human. Exp Cell Res 2021, 399(2): 112474.
- Tang JX, Xiao FH: Editorial: The regulation of proteostasis in aging. Front Cell Dev Biol 2023, 11: 1221510.
- Wang S, Long H, Hou L, Feng B, Ma Z, Wu Y, Zeng Y, Cai J, Zhang DW, Zhao G: The mitophagy pathway and its implications in human diseases. Signal Transduct Target Ther 2023, 8(1): 304.
- Liu L, Li Y, Chen G, Chen Q: Crosstalk between mitochondrial biogenesis and mitophagy to maintain mitochondrial homeostasis. J Biomed Sci 2023, 30(1): 86.
- Gomez CR: Role of heat shock proteins in aging and chronic inflammatory diseases. Geroscience 2021, 43(5): 2515-2532.
- Wyse C, O'Malley G, Coogan AN, McConkey S, Smith DJ: Seasonal and daytime variation in multiple immune parameters in humans: Evidence from 329,261 participants of the UK Biobank cohort. iScience 2021, 24(4): 102255.
- Rando TA, Wyss-Coray T: Asynchronous, contagious and digital aging. Nat Aging 2021, 1(1): 29-35.
- Guzik TJ, Skiba DS, Touyz RM, Harrison DG: The role of infiltrating immune cells in dysfunctional adipose tissue. Cardiovasc Res 2017, 113(9): 1009-1023.
- Wu Y, Wu C, Shi T, Cai Q, Wang T, Xiong Y, Zhang Y, Jiang W, Lu M, Chen Z, et al: FAP expression in adipose tissue macrophages promotes obesity and metabolic inflammation. Proc Natl Acad Sci U S A 2023, 120(51): e2303075120.
- Kawai T, Autieri MV, Scalia R: Adipose tissue inflammation and metabolic dysfunction in obesity. Am J Physiol Cell Physiol 2021, 320(3): C375-C391.
- Weinstock A, Brown EJ, Garabedian ML, Pena S, Sharma M, Lafaille J, Moore KJ, Fisher EA: Single-Cell RNA Sequencing of Visceral Adipose Tissue Leukocytes Reveals that Caloric Restriction Following Obesity Promotes the Accumulation of a Distinct Macrophage Population with Features of Phagocytic Cells. Immunometabolism 2019, 1: e190008.
- Di Rocco G, Trivisonno A, Trivisonno G, Toietta G: Dissecting human adipose tissue heterogeneity using single-cell omics technologies. Stem Cell Res Ther 2024, 15(1): 322.
- Uddin MS, Yu WS, Lim LW: Exploring ER stress response in cellular aging and neuroinflammation in Alzheimer's disease. Ageing Res Rev 2021, 70: 101417.
- Bordon Y: Targeting VCAM1 rejuvenates the brain in aged mice. Nat Rev Immunol 2019, 19(7): 415.
- Graja A, Garcia-Carrizo F, Jank AM, Gohlke S, Ambrosi TH, Jonas W, Ussar S, Kern M, Schürmann A, Aleksandrova K, et al: Loss of periostin occurs in aging adipose tissue of mice and its genetic ablation impairs adipose tissue lipid metabolism. Aging Cell 2018, 17(5): e12810.
- Kong DH, Kim YK, Kim MR, Jang JH, Lee S: Emerging Roles of Vascular Cell Adhesion Molecule-1 (VCAM-1) in Immunological Disorders and Cancer. Int J Mol Sci 2018, 19(4): 1057.
- Acosta-Rodríguez VA, Rijo-Ferreira F, Green CB, Takahashi JS: Importance of circadian timing for aging and longevity. Nat Commun 2021, 12(1): 2862.
- Xiao X, Feng H, Liao Y, Tang H, Li L, Li K, Hu F: Identification of key circadian rhythm genes in skin aging based on bioinformatics and machine learning. Aging (Albany NY) 2023, 15(20): 11672-11689.
- Malhan D, Yalçin M, Schoenrock B, Blottner D, Relógio A: Skeletal muscle gene expression dysregulation in long-term spaceflights and aging is clock-dependent. NPJ Microgravity 2023, 9(1): 30.
- Yuan P, Yang T, Mu J, Zhao J, Yang Y, Yan Z, Hou Y, Chen C, Xing J, Zhang H, et al: Circadian clock gene NPAS2 promotes reprogramming of glucose metabolism in hepatocellular carcinoma cells. Cancer Lett 2020, 469: 498-509.
- Yang T, Yuan P, Yang Y, Liang N, Wang Q, Li J, Lu R, Zhang H, Mu J, Yan Z, et al: NPAS2 Contributes to Liver Fibrosis by Direct Transcriptional Activation of Hes1 in Hepatic Stellate Cells. Mol Ther Nucleic Acids 2019, 18: 1009-1022.
- Xiong X, Lin Y, Lee J, Paul A, Yechoor V, Figueiro M, Ma K: Chronic circadian shift leads to adipose tissue inflammation and fibrosis. Mol Cell Endocrinol 2021, 521: 111110.
- Tenchov R, Sasso JM, Wang X, Zhou QA: Aging Hallmarks and Progression and Age-Related Diseases: A Landscape View of Research Advancement. ACS Chem Neurosci 2024, 15(1): 1-30.
- Palmer AK, Kirkland JL: Aging and adipose tissue: potential interventions for diabetes and regenerative medicine. Exp Gerontol 2016, 86: 97-105.
- Zhang K, Ma Y, Luo Y, Song Y, Xiong G, Ma Y, Sun X, Kan C: Metabolic diseases and healthy aging: identifying environmental and behavioral risk factors and promoting public health. Front Public Health 2023, 11: 1253506.
- Elliott ML, Caspi A, Houts RM, Ambler A, Broadbent JM, Hancox RJ, Harrington H, Hogan S, Keenan R, Knodt A, et al: Disparities in the pace of biological aging among midlife adults of the same chronological age have implications for future frailty risk and policy. Nat Aging 2021, 1(3): 295-308.
- Huang W, Hickson LJ, Eirin A, Kirkland JL, Lerman LO: Cellular senescence: the good, the bad and the unknown. Nat Rev Nephrol 2022, 18(10): 611-627.
- Martínez-Zamudio RI, Dewald HK, Vasilopoulos T, Gittens-Williams L, Fitzgerald-Bocarsly P, Herbig U: Senescence-associated β-galactosidase reveals the abundance of senescent CD8+ T cells in aging humans. Aging Cell 2021, 20(5): e13344.
- Foglia MJ, Poss KD: Building and re-building the heart by cardiomyocyte proliferation. Development 2016, 143(5): 729-740.
- Abdellatif M, Rainer PP, Sedej S, Kroemer G: Hallmarks of cardiovascular ageing. Nat Rev Cardiol 2023, 20(11): 754-777.
- Ou MY, Zhang H, Tan PC, Zhou SB, Li QF: Adipose tissue aging: mechanisms and therapeutic implications. Cell Death Dis 2022, 13(4): 300.
- Narasimhan A, Flores RR, Camell CD, Bernlohr DA, Robbins PD, Niedernhofer LJ: Cellular Senescence in Obesity and Associated Complications: a New Therapeutic Target. Curr Diab Rep 2022, 22(11): 537-548.
- Solhi M, Pirouzeh R, Zanjari N: Perspectives on healthy aging in middle age: Evidence for health promotion interventions. J Educ Health Promot 2022, 11: 5.
- Novais EJ, Tran VA, Johnston SN, Darris KR, Roupas AJ, Sessions GA, Shapiro IM, Diekman BO, Risbud MV: Long-term treatment with senolytic drugs Dasatinib and Quercetin ameliorates age-dependent intervertebral disc degeneration in mice. Nat Commun 2021, 12(1): 5213.
Asia-Pacific Journal of Surgical & Experimental Pathology
ISSN 2977-5817 (Online)
Copyright © Asia Pac J Surg Exp & Pathol. This
work is licensed under a Creative Commons AttributionNonCommercial-No Derivatives 4.0 International (CC BY-NC-ND 4.0)
License.