Review Article | Open Access
Aging-related comorbidities, life-style interventions and therapeutic strategies in heart failure
Nam Nhut Phan1, Fakhar-un-Nisa Yunus2
1Nguyen Tat Thanh University, Ho Chi Minh City, Vietnam is 298A Nguyen Tat Thanh, W.13, District 4, Ho Chi Minh city, Vietnam.
2Department of Zoology, Lahore College for Women University, Lahore, Pakistan.
Correspondence: Nam Nhut Phan (Nguyen Tat Thanh University, Ho Chi Minh City, Vietnam is 298A Nguyen Tat Thanh, W.13, District 4, Ho Chi Minh city, Vietnam; E-mail: NNphan@gmail.com).
Asia-Pacific Journal of Surgical & Experimental Pathology 2025, 2: 38-47. https://doi.org/10.32948/ajsep.2025.03.20
Received: 12 Jan 2025 | Accepted: 20 Mar 2025 | Published online: 30 Mar 2025
Key words heart failure, aging, aging-related comorbidities, exercise, anti-aging therapy
In the kidneys, aging leads to glomerular dysfunction, decreased renal blood flow, and compromised sodium handling, all of which contribute to the development of hypertension, an important risk factor for heart failure [31]. Systemic inflammation, oxidative damage, and imbalances in electrolytes in the kidneys accelerate the progression of heart failure [32]. The metabolic alterations in skeletal muscle due to aging also exacerbate heart failure. Altered secretion of myostatin (a myokine) adds to the complex network of metabolic and systemic changes associated with aging [33]. Accompanied by disrupted AMPK signaling and activation of the NF-κB pathway, sarcopenia impair mitochondrial function, reduce energy production, and promote systemic inflammation, all of which contribute to the development of cardiac diseases [34]. Reduced physical activity resulting from sarcopenia or muscle dysfunction exacerbates metabolic disturbances, accelerating the progression of heart failure [35]. Interestingly, the heart’s altered release of myostatin in chronic heart failure may stimulate proteolysis in an ActIIB/Smad-dependent manner, leading to skeletal muscle wasting [36]. In conclusion, the bidirectional communication between the heart and other organs underscores the impact of aging-related systemic decline during heart failure.
Aging-related development of coronary artery disease begins with the formation of atherosclerotic plaques, which can rupture and lead to thrombus formation (Figure 1, Table 1). The thrombus can obstruct the coronary arteries either partially or completely, resulting in myocardial ischemia, which is a key cause of heart failure [48, 49]. Ischemic conditions activate various molecular mechanisms, including the release of ATP and potassium ions due to cellular stress. These actions stimulate purinergic receptors and exacerbate inflammation. Simultaneously, the ischemic myocardium releases damage-associated molecular patterns that further intensify the inflammatory response [50, 51]. Chronic inflammation and oxidative stress significantly contribute to the progression of heart failure, promoting cardiomyocyte apoptosis, necrosis, and adverse cardiac remodeling. These processes are driven by hypertrophic signaling pathways, particularly those involving Akt and mTOR [52, 53]. The fibrotic response, regulated by transforming growth factor-beta (TGF-β) and collagen deposition, leads to increased myocardial stiffness. This ongoing stress, combined with maladaptive remodeling and dysfunction of the heart, ultimately results in heart failure [54]. Aging-related onset of hypertension put strain on both the heart and blood vessels, resulting in harmful structural and functional alterations that increase the risk of heart failure [55]. As individuals age, the regulation of the renin-angiotensin-aldosterone system (RAAS), a critical hormonal network involved in managing blood pressure, becomes disrupted. This disruption leads to an overproduction of angiotensin II, a powerful vasoconstrictor, which in turn triggers the hypertrophy of vascular smooth muscle cells, inflammation, and oxidative stress. These molecular processes contribute to endothelial dysfunction and are central to the development of hypertension [56]. Hypertension further drives detrimental changes in the heart through the activation of neurohormonal systems, particularly angiotensin II and the sympathetic nervous system. This activation initiates signaling pathways that promote cardiac hypertrophy, including the mitogen-activated protein kinase (MAPK) pathway, phosphoinositide 3-kinase (PI3K)/Akt signaling, p38-MAPK, and c-Jun N-terminal kinase (JNK) pathways, which lead to hypertrophy of cardiomyocytes and interstitial fibrosis [57].
Aging-related chronic kidney disease is characterized by a gradual decline in kidney function, including a reduced glomerular filtration rate and impaired sodium regulation, which creates a biochemical environment that accelerates the development of heart failure (Figure 1, Table 1) [58]. Dysfunction in sodium regulation leads to electrolyte imbalances, promoting sodium retention and fluid accumulation. This fluid retention activates the RAAS, resulting in the release of angiotensin II and aldosterone. Angiotensin II induces vasoconstriction, while aldosterone facilitates increased sodium and water reabsorption, together raising blood volume and systemic blood pressure, thereby imposing greater stress on the heart, and resulting in heart failure progression [59]. The accumulation of uremic toxins in advanced stages of chronic kidney disease contributes to heart failure through systemic inflammation and oxidative stress. These toxins impair endothelial function, reducing vasodilatory capacity and fostering a proinflammatory state that adversely affects cardiac health [60]. Elevated levels of cytokines, including IL-1β, IL-6, and TNF-α marks the chronic inflammatory milieu associated with chronic kidney disease, driving detrimental cardiac remodeling processes linked to heart failure [61]. In summary, aging-related obesity, diabetes, coronary artery disease, hypertension, and chronic kidney disease create a complex interplay of molecular, metabolic and inflammatory disturbances that accelerate the progression of heart failure.
Table 1. Comorbidities of aging exacerbate heart failure. |
|||
Comorbidity |
Key molecular mechanisms |
Systemic impact |
Ref. |
Obesity |
Insulin resistance, chronic inflammation, mitochondrial dysfunction, and oxidative stress. |
Promotes lipotoxicity, cardiac fibrosis, and energy imbalance. |
[37, 40-47] |
Diabetes |
Advanced glycation end-products, endothelial dysfunction, oxidative stress, and inflammation. |
Drives metabolic imbalance, cardiac fibrosis, and remodeling. |
[37-39] |
Coronary artery disease (CAD) |
Atherosclerosis, plaque rupture, chronic inflammation, and thrombus formation. |
Leads to ischemia, myocardial infarction, and heart failure. |
[48-54]
|
Hypertension |
RAAS activation, oxidative stress, and vascular inflammation. |
Causes cardiac hypertrophy, endothelial dysfunction, and fibrosis. |
[55-57]
|
Chronic kidney disease (CKD) |
Uremic toxin accumulation, fluid retention, and systemic inflammation. |
Contributes to hypertension, oxidative stress, and heart failure. |
[58-61]
|
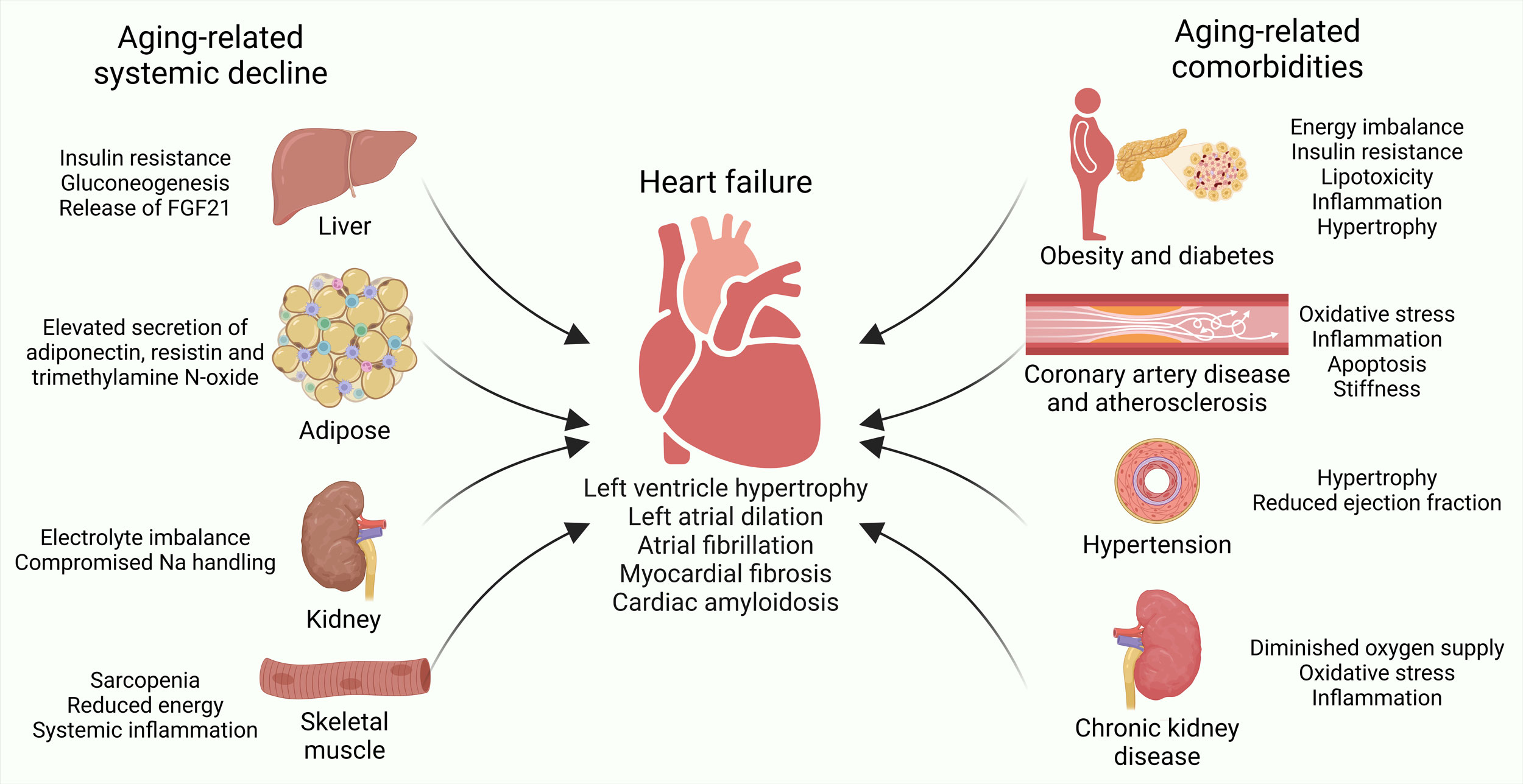
Physical activity, recognized for its antioxidative properties, may partially mitigate pathological cardiac remodeling in older adults by addressing several processes linked to age-related heart failure (Figure 2, Table 2). These include mitochondrial dysfunction, chronic inflammation, cellular aging (senescence), and reduced cardiomyocyte regeneration [8, 68, 69]. Studies have shown that aerobic exercise training, conducted over a duration of three to six months, enhances peak oxygen consumption and exercise efficiency in individuals aged 65 to 79 [70]. Engaging in progressive and intense endurance training can lead to physiological remodeling of the left ventricle in previously sedentary individuals over 65, characterized by an increase in ventricular mass while maintaining the mass-volume ratio [71]. Exercise has also been shown to promote mitochondrial biogenesis in the heart, improving energy metabolism and bolstering the antioxidant defense system in heart failure patients [72]. Physical activity also promotes cardiac autophagy, which, in turn, stimulates mitochondrial biogenesis, reduces local tissue inflammation, and improves heart function [72, 73]. Research shows that 21 days of voluntary running in mice increases telomerase activity and prevents cellular aging in the heart, demonstrating the geroprotective properties of regular exercise [74]. In aged myostatin knockout mice, cardiac structure and function are notably better preserved. Interestingly, exercise training reduces myostatin levels in the skeletal muscles of both chronic heart failure patients and animal models, highlighting the potential advantages of exercise in addressing age-related cardiac alterations [75]. Exercise protects against apoptotic cell death by suppressing the calcineurin/nuclear factor of activated T cells (NFAT) signaling pathway responsible for pathological hypertrophy, thereby preventing maladaptive remodeling in heart failure [76]. Moderate physical activity also reduces myocardial apoptosis and encourages cardiac regeneration through the activation of Janus kinase (JAK)/signal transducer and activator of transcription (STAT) signaling, pathways often suppressed in heart failure [77]. On a molecular level, exercise enhances SIRT3 expression in aging hearts, which helps mitigate mitochondrial stress [78]. Exercise also promotes the cardiac-specific production of insulin-like growth factor 1 (IGF-1), triggering PI3K/Akt signaling that fosters angiogenesis, reduces apoptosis and fibrosis, and supports physiological hypertrophy, ultimately improving cardiac performance in heart failure [79]. Exercise-induced cell division cycle 42 (Cdc42) functions as a negative modulator of physiological hypertrophy, limiting excessive heart enlargement. In Cdc42-deficient models, animals display exaggerated exercise-induced cardiac hypertrophy, which can progress to heart failure [80]. Aerobic exercise and combined training effectively reduce pro-inflammatory markers like IL-6 and TNF-α, addressing chronic inflammation in overweight heart failure patients [81]. These findings underscore the therapeutic potential of diet and exercise in heart failure management.
Table 2. Lifestyle interventions and therapeutic strategies to counteract aging-related heart failure. |
|||
Intervention/Strategy |
Mechanisms of action |
Clinical benefits |
Ref. |
Plant-based diet |
Improves metabolic balance, reduces inflammation, and enhances ketone utilization. |
Lowers heart failure risk and improves overall heart health. |
[62, 63] |
Caloric restriction |
Reduces adiposity, improves metabolic efficiency, and supports cardiac function. |
Benefits overweight patients; not suitable for underweight individuals. |
[64] |
Micronutrient supplementation |
Provides essential nutrients (e.g., iron, omega-3 fatty acids) to improve heart efficiency and function. |
Reduces hospitalization and improves ejection fraction. |
[65-67] |
Physical activity |
Enhances mitochondrial biogenesis, reduces inflammation, promotes autophagy, and boosts energy metabolism. |
Improves cardiac function and reduces pathological remodeling. |
[8, 68-81] |
Senolytics (e.g., Dasatinib and Quercetin) |
Targets and clears senescent cells, reduces fibrosis, and enhances cardiomyocyte regeneration. |
Improves cardiac function and lifespan. |
[83-88] |
Sirtuin activators (e.g., Resveratrol) |
Activates mitochondrial pathways, reduces oxidative stress, and supports endothelial function. |
Enhances heart health and promotes longevity. |
[9, 90-94] |
SGLT2 inhibitors |
Activates autophagy and reduces oxidative stress through AMPK/SIRT1 pathways. |
Reduces hospitalizations and mortality in diabetic patients. |
[95-98] |
Anti-inflammatory agents |
Reduces systemic and cardiac inflammation via cytokine modulation (e.g., IL-1 blockers, Methotrexate). |
Prevents cardiac remodeling and improves exercise capacity. |
[99-105] |
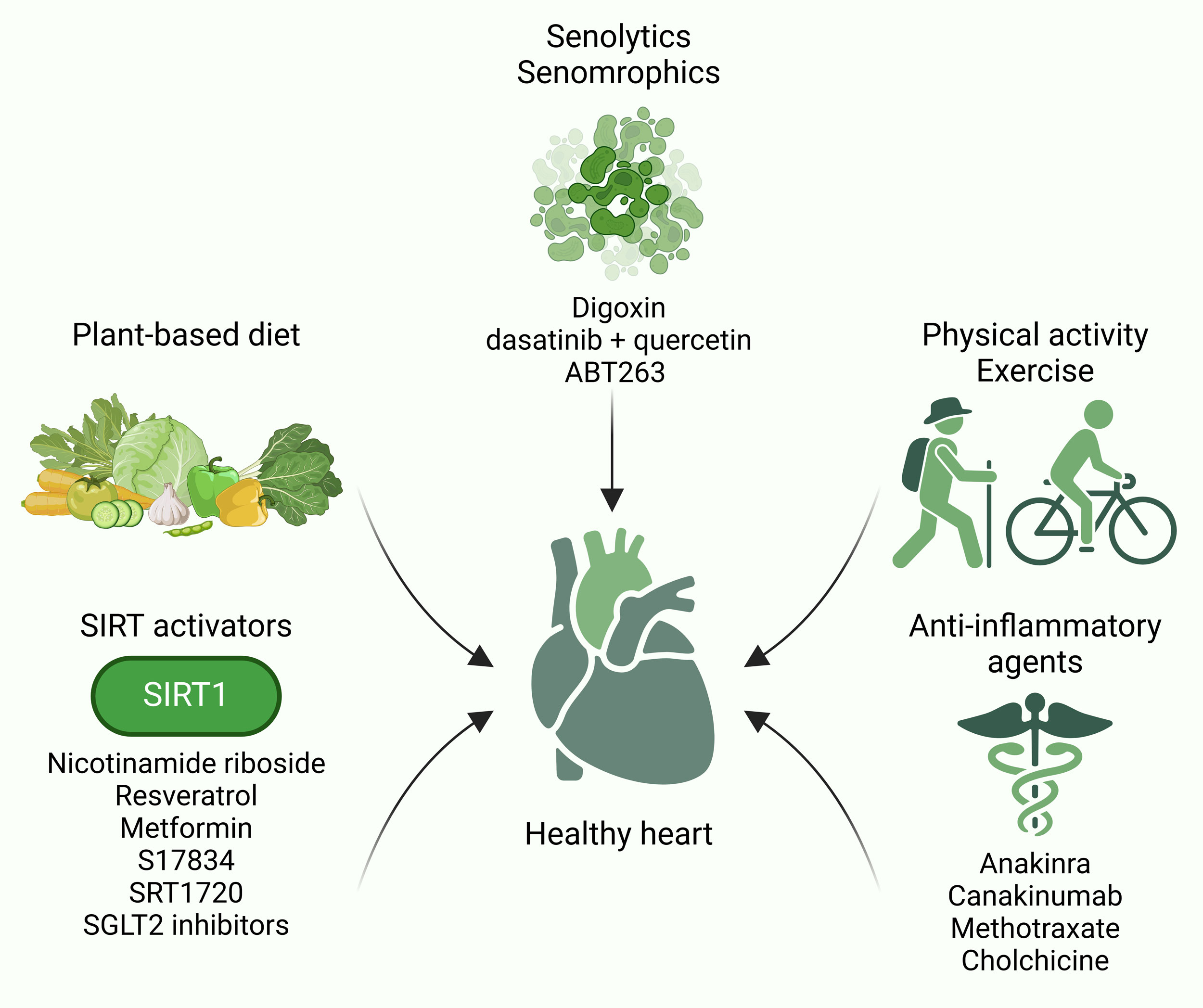
In human hearts, a decline in NAD+ levels is commonly observed with aging [89]. NAD+ is essential as it is the precursor for several enzymes, including SIRTs, which are vital for managing cardiomyocyte survival and mitochondrial membrane stability via histone deacetylation. Restoring NAD+ levels using nicotinamide riboside supplementation holds promise as a strategy to combat the effects of cardiac aging and to mitigate cardiovascular diseases, including heart failure (Figure 2, Table 2) [90]. A study administering 1,000 mg of nicotinamide riboside twice a day to clinically stable heart failure patients demonstrated its safety and tolerability, as well as its ability to nearly double NAD+ levels in whole blood [91]. Resveratrol, known to activate SIRT1, holds promise in alleviating oxidative and inflammatory stress. It offers several vascular health benefits, such as reducing platelet aggregation and arterial stiffness, and protecting against conditions like atherosclerosis, hypertension, ischemia/reperfusion injury, and heart failure [9]. Metformin, which activates autophagy through SIRT1, has been shown to decrease the incidence and mortality of heart failure in individuals with cardiovascular diseases [92]. S17834, a SIRT1 activator, also demonstrates anti-inflammatory and anti-atherogenic properties in models of accelerated cardiovascular aging [93], SRT1720, another SIRT1 activator, has been shown to enhance endothelial function, decrease vascular oxidative stress and inflammation, and inhibit plaque formation associated with atherosclerosis, thereby contributing to improved health and lifespan in accelerated aging models [94]. Sodium-glucose cotransporter 2 (SGLT2) inhibitors, primarily used for managing blood glucose levels in diabetes, promote autophagy by activating the AMPK/SIRT1 pathway [95]. Treatment with SGLT2 inhibitors in diabetic patients have shown a reduction in cardiovascular mortality and hospitalizations related to heart failure [96-98].
Targeting IL-1β, a significant pro-inflammatory cytokine, has been shown to offer cardioprotective effects by reducing inflammation and enhancing cardiac function in heart failure (Figure 2, Table 2) [99]. Anakinra (an IL-1 blocker) treatment in heart failure patients with high plasma C-reactive protein (CRP) levels significantly improved the systemic inflammation and increased aerobic exercise capacity [100]. Canakinumab (an IL-1β inhibitor) treatment in patients with myocardial infarction and elevated CRP substantial reduced both heart failure-related hospitalizations and mortality risk [101]. Methotrexate, an established anti-rheumatic agent, offers cardiovascular benefits by curbing inflammation, reducing atherosclerosis, and preventing vascular aging. It has also been linked to a lower risk of heart failure in rheumatoid arthritis patients and can improve endothelial function, arterial stiffness, and hypertension [102, 103]. Colchicine, a drug traditionally used to treat gout by blocking NLRP3 inflammasome activation, has recently been recognized for its potential cardiovascular benefits, including anti-aging effects. Preclinical studies suggest that colchicine improves exercise capacity and mitigates cardiac diastolic dysfunction, oxidative stress, and fibrosis in a hypertensive heart failure mouse model [104]. However, a prospective, randomized trial involving stable chronic heart failure patients found that while colchicine effectively reduced inflammatory markers, it did not significantly impact functional status or the risk of death or hospitalization due to heart failure [105].
In summary, despite encouraging findings, uncertainties persist regarding the role of above discussed aging-targeted therapies (senomorphics, senolytics, SIRT-activating and anti-inflammatory agents) against heart failure. Hence, comprehensive long-term clinical studies are necessary to confirm the enduring benefits of these therapies in maintaining cardiac function, and alleviating heart failure.
No applicable.
Ethics approval
No applicable.
Data availability
This narrative review is based on previously published studies and publicly available data. No new datasets were generated or analyzed for the current review.
Funding
None.
Authors’ contribution
Nam Nhut Phan contributed to the design, writing of this review article and final submission online. Fakhar-un-Nisa Yunus cellected data and drew figures for the manuscript.
Competing interests
The authors declare no competing interests.
- Roger VL: Epidemiology of Heart Failure: A Contemporary Perspective. Circ Res 2021, 128(10): 1421-1434.
- Arrigo M, Jessup M, Mullens W, Reza N, Shah AM, Sliwa K, Mebazaa A: Acute heart failure. Nat Rev Dis Primers 2020, 6(1): 16.
- Amorim JA, Coppotelli G, Rolo AP, Palmeira CM, Ross JM, Sinclair DA: Mitochondrial and metabolic dysfunction in ageing and age-related diseases. Nat Rev Endocrinol 2022, 18(4): 243-258.
- Takada S, Sabe H, Kinugawa S: Abnormalities of Skeletal Muscle, Adipocyte Tissue, and Lipid Metabolism in Heart Failure: Practical Therapeutic Targets. Front Cardiovasc Med 2020, 7: 79.
- Screever EM, van der Wal MHL, van Veldhuisen DJ, Jaarsma T, Koops A, van Dijk KS, Warink-Riemersma J, Coster JE, Westenbrink BD, van der Meer P, et al: Comorbidities complicating heart failure: changes over the last 15 years. Clin Res Cardiol 2023, 112(1): 123-133.
- López-Otín C, Blasco MA, Partridge L, Serrano M, Kroemer G: Hallmarks of aging: An expanding universe. Cell 2023, 186(2): 243-278.
- Li H, Hastings MH, Rhee J, Trager LE, Roh JD, Rosenzweig A: Targeting Age-Related Pathways in Heart Failure. Circ Res 2020, 126(4): 533-551.
- Roh J, Rhee J, Chaudhari V, Rosenzweig A: The Role of Exercise in Cardiac Aging: From Physiology to Molecular Mechanisms. Circ Res 2016, 118(2): 279-295.
- Alfaras I, Di Germanio C, Bernier M, Csiszar A, Ungvari Z, Lakatta EG, de Cabo R: Pharmacological Strategies to Retard Cardiovascular Aging. Circ Res 2016, 118(10): 1626-1642.
- Liu E, Lampert BC: Heart Failure in Older Adults: Medical Management and Advanced Therapies. Geriatrics (Basel) 2022, 7(2): 36.
- Cheng S, Fernandes VR, Bluemke DA, McClelland RL, Kronmal RA, Lima JA: Age-related left ventricular remodeling and associated risk for cardiovascular outcomes: the Multi-Ethnic Study of Atherosclerosis. Circ Cardiovasc Imaging 2009, 2(3): 191-198.
- Nayor M, Cooper LL, Enserro DM, Xanthakis V, Larson MG, Benjamin EJ, Aragam J, Mitchell GF, Vasan RS: Left Ventricular Diastolic Dysfunction in the Community: Impact of Diagnostic Criteria on the Burden, Correlates, and Prognosis. J Am Heart Assoc 2018, 7(11): e008291.
- Christou DD, Seals DR: Decreased maximal heart rate with aging is related to reduced {beta}-adrenergic responsiveness but is largely explained by a reduction in intrinsic heart rate. J Appl Physiol (1985) 2008, 105(1): 24-29.
- Wolsk E, Bakkestrøm R, Thomsen JH, Balling L, Andersen MJ, Dahl JS, Hassager C, Møller JE, Gustafsson F: The Influence of Age on Hemodynamic Parameters During Rest and Exercise in Healthy Individuals. JACC Heart Fail 2017, 5(5): 337-346.
- Tomás MT, Galán-Mercant A, Carnero EA, Fernandes B: Functional Capacity and Levels of Physical Activity in Aging: A 3-Year Follow-up. Front Med (Lausanne) 2017, 4: 244.
- Yeh CH, Chen CY, Kuo YE, Chen CW, Kuo TBJ, Kuo KL, Chen HM, Huang HY, Chern CM, Yang CCH: Role of the autonomic nervous system in young, middle-aged, and older individuals with essential hypertension and sleep-related changes in neurocardiac regulation. Sci Rep 2023, 13(1): 22623.
- Rostagno C: Heart valve disease in elderly. World J Cardiol 2019, 11(2): 71-83.
- Evin M, Redheuil A, Soulat G, Perdrix L, Ashrafpoor G, Giron A, Lamy J, Defrance C, Roux C, Hatem SN, et al: Left atrial aging: a cardiac magnetic resonance feature-tracking study. Am J Physiol Heart Circ Physiol 2016, 310(5): H542-549.
- Gao P, Gao X, Xie B, Tse G, Liu T: Aging and atrial fibrillation: A vicious circle. Int J Cardiol 2024, 395: 131445.
- Haddad F, Hunt SA, Rosenthal DN, Murphy DJ: Right ventricular function in cardiovascular disease, part I: Anatomy, physiology, aging, and functional assessment of the right ventricle. Circulation 2008, 117(11): 1436-1448.
- Wehrum T, Lodemann T, Hagenlocher P, Stuplich J, Ngo BTT, Grundmann S, Hennemuth A, Hennig J, Harloff A: Age-related changes of right atrial morphology and inflow pattern assessed using 4D flow cardiovascular magnetic resonance: results of a population-based study. J Cardiovasc Magn Reson 2018, 20(1): 38.
- Li G, Yang J, Zhang D, Wang X, Han J, Guo X: Research Progress of Myocardial Fibrosis and Atrial Fibrillation. Front Cardiovasc Med 2022, 9: 889706.
- Wu D, Chen W: Molecular mechanisms and emerging therapies in wild-type transthyretin amyloid cardiomyopathy. Heart Fail Rev 2024, 29(2): 511-521.
- Onyango AN: Excessive gluconeogenesis causes the hepatic insulin resistance paradox and its sequelae. Heliyon 2022, 8(12): e12294.
- Tan H, Yue T, Chen Z, Wu W, Xu S, Weng J: Targeting FGF21 in cardiovascular and metabolic diseases: from mechanism to medicine. Int J Biol Sci 2023, 19(1): 66-88.
- Zsombok A, Desmoulins LD, Derbenev AV: Sympathetic circuits regulating hepatic glucose metabolism: where we stand. Physiol Rev 2024, 104(1): 85-101.
- Li C, Sun XN, Zhao S, Scherer PE: Crosstalk Between Adipose Tissue and the Heart: An Update. J Transl Int Med 2022, 10(3): 219-226.
- Beatty AL, Zhang MH, Ku IA, Na B, Schiller NB, Whooley MA: Adiponectin is associated with increased mortality and heart failure in patients with stable ischemic heart disease: data from the Heart and Soul Study. Atherosclerosis 2012, 220(2): 587-592.
- Zhao B, Bouchareb R, Lebeche D: Resistin deletion protects against heart failure injury by targeting DNA damage response. Cardiovasc Res 2022, 118(8): 1947-1963.
- Yoshida Y, Shimizu I, Shimada A, Nakahara K, Yanagisawa S, Kubo M, Fukuda S, Ishii C, Yamamoto H, Ishikawa T, et al: Brown adipose tissue dysfunction promotes heart failure via a trimethylamine N-oxide-dependent mechanism. Sci Rep 2022, 12(1): 14883.
- Mullens W, Damman K, Testani JM, Martens P, Mueller C, Lassus J, Tang WHW, Skouri H, Verbrugge FH, Orso F, et al: Evaluation of kidney function throughout the heart failure trajectory - a position statement from the Heart Failure Association of the European Society of Cardiology. Eur J Heart Fail 2020, 22(4): 584-603.
- Cardozo L, Borges NA, Ribeiro M, Yee-Moon Wang A, Mafra D: Protect the Kidneys and Save the Heart Using the Concept of Food as Medicine. J Ren Nutr 2023, 33(6s): S110-S117.
- Balakrishnan R, Thurmond DC: Mechanisms by Which Skeletal Muscle Myokines Ameliorate Insulin Resistance. Int J Mol Sci 2022, 23(9): 4636.
- Larsson L, Degens H, Li M, Salviati L, Lee YI, Thompson W, Kirkland JL, Sandri M: Sarcopenia: Aging-Related Loss of Muscle Mass and Function. Physiol Rev 2019, 99(1): 427-511.
- Curcio F, Testa G, Liguori I, Papillo M, Flocco V, Panicara V, Galizia G, Della-Morte D, Gargiulo G, Cacciatore F, et al: Sarcopenia and Heart Failure. Nutrients 2020, 12(1): 211.
- Knapp M, Supruniuk E, Górski J: Myostatin and the Heart. Biomolecules 2023, 13(12): 1777.
- Elendu C, Amaechi DC, Elendu TC, Ashna M, Ross-Comptis J, Ansong SO, Egbunu EO, Okafor GC, Jingwa KA, Akintunde AA, et al: Heart failure and diabetes: Understanding the bidirectional relationship. Medicine (Baltimore) 2023, 102(37): e34906.
- Ormazabal V, Nair S, Elfeky O, Aguayo C, Salomon C, Zuñiga FA: Association between insulin resistance and the development of cardiovascular disease. Cardiovasc Diabetol 2018, 17(1): 122.
- González P, Lozano P, Ros G, Solano F: Hyperglycemia and Oxidative Stress: An Integral, Updated and Critical Overview of Their Metabolic Interconnections. Int J Mol Sci 2023, 24(11): 9352.
- Cavaliere G, Cimmino F, Trinchese G, Catapano A, Petrella L, D'Angelo M, Lucchin L, Mollica MP: From Obesity-Induced Low-Grade Inflammation to Lipotoxicity and Mitochondrial Dysfunction: Altered Multi-Crosstalk between Adipose Tissue and Metabolically Active Organs. Antioxidants (Basel) 2023, 12(6): 1172.
- Hasheminasabgorji E, Jha JC: Dyslipidemia, Diabetes and Atherosclerosis: Role of Inflammation and ROS-Redox-Sensitive Factors. Biomedicines 2021, 9(11): 1602.
- Smail HO: The epigenetics of diabetes, obesity, overweight and cardiovascular disease. AIMS Genet 2019, 6(3): 36-45.
- Battineni G, Sagaro GG, Chintalapudi N, Amenta F, Tomassoni D, Tayebati SK: Impact of Obesity-Induced Inflammation on Cardiovascular Diseases (CVD). Int J Mol Sci 2021, 22(9): 4798.
- Manna P, Jain SK: Obesity, Oxidative Stress, Adipose Tissue Dysfunction, and the Associated Health Risks: Causes and Therapeutic Strategies. Metab Syndr Relat Disord 2015, 13(10): 423-444.
- Cavalera M, Wang J, Frangogiannis NG: Obesity, metabolic dysfunction, and cardiac fibrosis: pathophysiological pathways, molecular mechanisms, and therapeutic opportunities. Transl Res 2014, 164(4): 323-335.
- Ebong IA, Goff DC, Jr., Rodriguez CJ, Chen H, Bertoni AG: Mechanisms of heart failure in obesity. Obes Res Clin Pract 2014, 8(6): e540-8.
- Berezin AE, Berezin AA, Lichtenauer M: Emerging Role of Adipocyte Dysfunction in Inducing Heart Failure Among Obese Patients With Prediabetes and Known Diabetes Mellitus. Front Cardiovasc Med 2020, 7: 583175.
- Frostegård J: Immunity, atherosclerosis and cardiovascular disease. BMC Med 2013, 11: 117.
- Kong P, Cui ZY, Huang XF, Zhang DD, Guo RJ, Han M: Inflammation and atherosclerosis: signaling pathways and therapeutic intervention. Signal Transduct Target Ther 2022, 7(1): 131.
- Severino P, D'Amato A, Pucci M, Infusino F, Birtolo LI, Mariani MV, Lavalle C, Maestrini V, Mancone M, Fedele F: Ischemic Heart Disease and Heart Failure: Role of Coronary Ion Channels. Int J Mol Sci 2020, 21(9): 3167.
- Schädlich IS, Winzer R, Stabernack J, Tolosa E, Magnus T, Rissiek B: The role of the ATP-adenosine axis in ischemic stroke. Semin Immunopathol 2023, 45(3): 347-365.
- Aoyagi T, Matsui T: Phosphoinositide-3 kinase signaling in cardiac hypertrophy and heart failure. Curr Pharm Des 2011, 17(18): 1818-1824.
- Sciarretta S, Forte M, Frati G, Sadoshima J: New Insights Into the Role of mTOR Signaling in the Cardiovascular System. Circ Res 2018, 122(3): 489-505.
- Sweeney M, Corden B, Cook SA: Targeting cardiac fibrosis in heart failure with preserved ejection fraction: mirage or miracle? EMBO Mol Med 2020, 12(10): e10865.
- Masenga SK, Kirabo A: Hypertensive heart disease: risk factors, complications and mechanisms. Front Cardiovasc Med 2023, 10: 1205475.
- Cabandugama PK, Gardner MJ, Sowers JR: The Renin Angiotensin Aldosterone System in Obesity and Hypertension: Roles in the Cardiorenal Metabolic Syndrome. Med Clin North Am 2017, 101(1): 129-137.
- Forrester SJ, Booz GW, Sigmund CD, Coffman TM, Kawai T, Rizzo V, Scalia R, Eguchi S: Angiotensin II Signal Transduction: An Update on Mechanisms of Physiology and Pathophysiology. Physiol Rev 2018, 98(3): 1627-1738.
- Romagnani P, Remuzzi G, Glassock R, Levin A, Jager KJ, Tonelli M, Massy Z, Wanner C, Anders HJ: Chronic kidney disease. Nat Rev Dis Primers 2017, 3: 17088.
- Mehta JK, Kaur G, Buttar HS, Bagabir HA, Bagabir RA, Bagabir SA, Haque S, Tuli HS, Telessy IG: Role of the renin-angiotensin system in the pathophysiology of coronary heart disease and heart failure: Diagnostic biomarkers and therapy with drugs and natural products. Front Physiol 2023, 14: 1034170.
- Pieniazek A, Bernasinska-Slomczewska J, Gwozdzinski L: Uremic Toxins and Their Relation with Oxidative Stress Induced in Patients with CKD. Int J Mol Sci 2021, 22(12): 6196.
- Colombo PC, Ganda A, Lin J, Onat D, Harxhi A, Iyasere JE, Uriel N, Cotter G: Inflammatory activation: cardiac, renal, and cardio-renal interactions in patients with the cardiorenal syndrome. Heart Fail Rev 2012, 17(2): 177-190.
- Lara KM, Levitan EB, Gutierrez OM, Shikany JM, Safford MM, Judd SE, Rosenson RS: Dietary Patterns and Incident Heart Failure in U.S. Adults Without Known Coronary Disease. J Am Coll Cardiol 2019, 73(16): 2036-2045.
- Bedi KC, Jr., Snyder NW, Brandimarto J, Aziz M, Mesaros C, Worth AJ, Wang LL, Javaheri A, Blair IA, Margulies KB, et al: Evidence for Intramyocardial Disruption of Lipid Metabolism and Increased Myocardial Ketone Utilization in Advanced Human Heart Failure. Circulation 2016, 133(8): 706-716.
- Bianchi VE: Caloric restriction in heart failure: A systematic review. Clin Nutr ESPEN 2020, 38: 50-60.
- Tkaczyszyn M, Fudim M, Ponikowski P, Biegus J: Pathophysiology and Treatment Opportunities of Iron Deficiency in Heart Failure: Is There a Need for Further Trials? Curr Heart Fail Rep 2023, 20(4): 300-307.
- Nodari S, Triggiani M, Campia U, Manerba A, Milesi G, Cesana BM, Gheorghiade M, Dei Cas L: Effects of n-3 polyunsaturated fatty acids on left ventricular function and functional capacity in patients with dilated cardiomyopathy. J Am Coll Cardiol 2011, 57(7): 870-879.
- Lombardi M, Carbone S, Del Buono MG, Chiabrando JG, Vescovo GM, Camilli M, Montone RA, Vergallo R, Abbate A, Biondi-Zoccai G, et al: Omega-3 fatty acids supplementation and risk of atrial fibrillation: an updated meta-analysis of randomized controlled trials. Eur Heart J Cardiovasc Pharmacother 2021, 7(4): e69-e70.
- Danduboyina A, Panjiyar BK, Borra SR, Panicker SS: Cardiovascular Benefits of Resistance Training in Patients With Heart Failure With Reduced Ejection Fraction: A Systematic Review. Cureus 2023, 15(10): e47813.
- Isath A, Koziol KJ, Martinez MW, Garber CE, Martinez MN, Emery MS, Baggish AL, Naidu SS, Lavie CJ, Arena R, et al: Exercise and cardiovascular health: A state-of-the-art review. Prog Cardiovasc Dis 2023, 79: 44-52.
- Woo JS, Derleth C, Stratton JR, Levy WC: The influence of age, gender, and training on exercise efficiency. J Am Coll Cardiol 2006, 47(5): 1049-1057.
- Fujimoto N, Prasad A, Hastings JL, Arbab-Zadeh A, Bhella PS, Shibata S, Palmer D, Levine BD: Cardiovascular effects of 1 year of progressive and vigorous exercise training in previously sedentary individuals older than 65 years of age. Circulation 2010, 122(18): 1797-1805.
- Campos JC, Queliconi BB, Bozi LHM, Bechara LRG, Dourado PMM, Andres AM, Jannig PR, Gomes KMS, Zambelli VO, Rocha-Resende C, et al: Exercise reestablishes autophagic flux and mitochondrial quality control in heart failure. Autophagy 2017, 13(8): 1304-1317.
- Bernardo BC, Ooi JYY, Weeks KL, Patterson NL, McMullen JR: Understanding Key Mechanisms of Exercise-Induced Cardiac Protection to Mitigate Disease: Current Knowledge and Emerging Concepts. Physiol Rev 2018, 98(1): 419-475.
- Werner C, Hanhoun M, Widmann T, Kazakov A, Semenov A, Pöss J, Bauersachs J, Thum T, Pfreundschuh M, Müller P, et al: Effects of physical exercise on myocardial telomere-regulating proteins, survival pathways, and apoptosis. J Am Coll Cardiol 2008, 52(6): 470-482.
- Lenk K, Erbs S, Höllriegel R, Beck E, Linke A, Gielen S, Winkler SM, Sandri M, Hambrecht R, Schuler G, et al: Exercise training leads to a reduction of elevated myostatin levels in patients with chronic heart failure. Eur J Prev Cardiol 2012, 19(3): 404-411.
- Oliveira RS, Ferreira JC, Gomes ER, Paixão NA, Rolim NP, Medeiros A, Guatimosim S, Brum PC: Cardiac anti-remodelling effect of aerobic training is associated with a reduction in the calcineurin/NFAT signalling pathway in heart failure mice. J Physiol 2009, 587(Pt 15): 3899-3910.
- Pahlavani HA: Exercise-induced signaling pathways to counteracting cardiac apoptotic processes. Front Cell Dev Biol 2022, 10: 950927.
- Zhao D, Sun Y, Tan Y, Zhang Z, Hou Z, Gao C, Feng P, Zhang X, Yi W, Gao F: Short-Duration Swimming Exercise after Myocardial Infarction Attenuates Cardiac Dysfunction and Regulates Mitochondrial Quality Control in Aged Mice. Oxid Med Cell Longev 2018, 2018: 4079041.
- Bass-Stringer S, Tai CMK, McMullen JR: IGF1-PI3K-induced physiological cardiac hypertrophy: Implications for new heart failure therapies, biomarkers, and predicting cardiotoxicity. J Sport Health Sci 2021, 10(6): 637-647.
- Maillet M, Lynch JM, Sanna B, York AJ, Zheng Y, Molkentin JD: Cdc42 is an antihypertrophic molecular switch in the mouse heart. J Clin Invest 2009, 119(10): 3079-3088.
- Malandish A, Gulati M: The impacts of exercise interventions on inflammaging markers in overweight/obesity patients with heart failure: A systematic review and meta-analysis of randomized controlled trials. Int J Cardiol Heart Vasc 2023, 47: 101234.
- Baker DJ, Childs BG, Durik M, Wijers ME, Sieben CJ, Zhong J, Saltness RA, Jeganathan KB, Verzosa GC, Pezeshki A, et al: Naturally occurring p16(Ink4a)-positive cells shorten healthy lifespan. Nature 2016, 530(7589): 184-189.
- Lewis-McDougall FC, Ruchaya PJ, Domenjo-Vila E, Shin Teoh T, Prata L, Cottle BJ, Clark JE, Punjabi PP, Awad W, Torella D, et al: Aged-senescent cells contribute to impaired heart regeneration. Aging Cell 2019, 18(3): e12931.
- Lee H, Wilson D, Bunting KV, Kotecha D, Jackson T: Repurposing digoxin for geroprotection in patients with frailty and multimorbidity. Ageing Res Rev 2023, 86: 101860.
- Zhu Y, Tchkonia T, Pirtskhalava T, Gower AC, Ding H, Giorgadze N, Palmer AK, Ikeno Y, Hubbard GB, Lenburg M, et al: The Achilles' heel of senescent cells: from transcriptome to senolytic drugs. Aging Cell 2015, 14(4): 644-658.
- Yu S, Kim SR, Jiang K, Ogrodnik M, Zhu XY, Ferguson CM, Tchkonia T, Lerman A, Kirkland JL, Lerman LO: Quercetin Reverses Cardiac Systolic Dysfunction in Mice Fed with a High-Fat Diet: Role of Angiogenesis. Oxid Med Cell Longev 2021, 2021: 8875729.
- Anderson R, Lagnado A, Maggiorani D, Walaszczyk A, Dookun E, Chapman J, Birch J, Salmonowicz H, Ogrodnik M, Jurk D, et al: Length-independent telomere damage drives post-mitotic cardiomyocyte senescence. Embo j 2019, 38(5): e100492.
- Jia K, Dai Y, Liu A, Li X, Wu L, Lu L, Bao Y, Jin Q: Senolytic Agent Navitoclax Inhibits Angiotensin II-Induced Heart Failure in Mice. J Cardiovasc Pharmacol 2020, 76(4): 452-460.
- Lee CF, Chavez JD, Garcia-Menendez L, Choi Y, Roe ND, Chiao YA, Edgar JS, Goo YA, Goodlett DR, Bruce JE, et al: Normalization of NAD+ Redox Balance as a Therapy for Heart Failure. Circulation 2016, 134(12): 883-894.
- Yuan Y, Liang B, Liu XL, Liu WJ, Huang BH, Yang SB, Gao YZ, Meng JS, Li MJ, Ye T, et al: Targeting NAD+: is it a common strategy to delay heart aging? Cell Death Discov 2022, 8(1): 230.
- Wang DD, Airhart SE, Zhou B, Shireman LM, Jiang S, Melendez Rodriguez C, Kirkpatrick JN, Shen DD, Tian R, O'Brien KD: Safety and Tolerability of Nicotinamide Riboside in Heart Failure With Reduced Ejection Fraction. JACC Basic Transl Sci 2022, 7(12): 1183-1196.
- Li T, Providencia R, Jiang W, Liu M, Yu L, Gu C, Chang ACY, Ma H: Association of Metformin with the Mortality and Incidence of Cardiovascular Events in Patients with Pre-existing Cardiovascular Diseases. Drugs 2022, 82(3): 311-322.
- Xu S, Jiang B, Hou X, Shi C, Bachschmid MM, Zang M, Verbeuren TJ, Cohen RA: High-fat diet increases and the polyphenol, S17834, decreases acetylation of the sirtuin-1-dependent lysine-382 on p53 and apoptotic signaling in atherosclerotic lesion-prone aortic endothelium of normal mice. J Cardiovasc Pharmacol 2011, 58(3): 263-271.
- Gano LB, Donato AJ, Pasha HM, Hearon CM, Jr., Sindler AL, Seals DR: The SIRT1 activator SRT1720 reverses vascular endothelial dysfunction, excessive superoxide production, and inflammation with aging in mice. Am J Physiol Heart Circ Physiol 2014, 307(12): H1754-1763.
- Packer M: Autophagy-dependent and -independent modulation of oxidative and organellar stress in the diabetic heart by glucose-lowering drugs. Cardiovasc Diabetol 2020, 19(1): 62.
- Zinman B, Wanner C, Lachin JM, Fitchett D, Bluhmki E, Hantel S, Mattheus M, Devins T, Johansen OE, Woerle HJ, et al: Empagliflozin, Cardiovascular Outcomes, and Mortality in Type 2 Diabetes. N Engl J Med 2015, 373(22): 2117-2128.
- Neal B, Perkovic V, Mahaffey KW, de Zeeuw D, Fulcher G, Erondu N, Shaw W, Law G, Desai M, Matthews DR: Canagliflozin and Cardiovascular and Renal Events in Type 2 Diabetes. N Engl J Med 2017, 377(7): 644-657.
- Wiviott SD, Raz I, Bonaca MP, Mosenzon O, Kato ET, Cahn A, Silverman MG, Zelniker TA, Kuder JF, Murphy SA, et al: Dapagliflozin and Cardiovascular Outcomes in Type 2 Diabetes. N Engl J Med 2019, 380(4): 347-357.
- Van Tassell BW, Seropian IM, Toldo S, Mezzaroma E, Abbate A: Interleukin-1β induces a reversible cardiomyopathy in the mouse. Inflamm Res 2013, 62(7): 637-640.
- Van Tassell BW, Arena R, Biondi-Zoccai G, Canada JM, Oddi C, Abouzaki NA, Jahangiri A, Falcao RA, Kontos MC, Shah KB, et al: Effects of interleukin-1 blockade with anakinra on aerobic exercise capacity in patients with heart failure and preserved ejection fraction (from the D-HART pilot study). Am J Cardiol 2014, 113(2): 321-327.
- Everett BM, Cornel JH, Lainscak M, Anker SD, Abbate A, Thuren T, Libby P, Glynn RJ, Ridker PM: Anti-Inflammatory Therapy With Canakinumab for the Prevention of Hospitalization for Heart Failure. Circulation 2019, 139(10): 1289-1299.
- Mangoni AA, Tommasi S, Zinellu A, Sotgia S, Bassu S, Piga M, Erre GL, Carru C: Methotrexate and Vasculoprotection: Mechanistic Insights and Potential Therapeutic Applications in Old Age. Curr Pharm Des 2019, 25(39): 4175-4184.
- Ahlers MJ, Lowery BD, Farber-Eger E, Wang TJ, Bradham W, Ormseth MJ, Chung CP, Stein CM, Gupta DK: Heart Failure Risk Associated With Rheumatoid Arthritis-Related Chronic Inflammation. J Am Heart Assoc 2020, 9(10): e014661.
- Shen S, Duan J, Hu J, Qi Y, Kang L, Wang K, Chen J, Wu X, Xu B, Gu R: Colchicine alleviates inflammation and improves diastolic dysfunction in heart failure rats with preserved ejection fraction. Eur J Pharmacol 2022, 929: 175126.
- Deftereos S, Giannopoulos G, Panagopoulou V, Bouras G, Raisakis K, Kossyvakis C, Karageorgiou S, Papadimitriou C, Vastaki M, Kaoukis A, et al: Anti-inflammatory treatment with colchicine in stable chronic heart failure: a prospective, randomized study. JACC Heart Fail 2014, 2(2): 131-137.
- Clayton ZS, Craighead DH, Darvish S, Coppock M, Ludwig KR, Brunt VE, Seals DR, Rossman MJ: Promoting healthy cardiovascular aging: emerging topics. J Cardiovasc Aging 2022, 2: 43.
- Ibrahim NE, Gaggin HK, Konstam MA, Januzzi JL, Jr.: Established and Emerging Roles of Biomarkers in Heart Failure Clinical Trials. Circ Heart Fail 2016, 9(9): e002528.
- Bavishi A, Patel RB: Addressing Comorbidities in Heart Failure: Hypertension, Atrial Fibrillation, and Diabetes. Heart Fail Clin 2020, 16(4): 441-456.
Asia-Pacific Journal of Surgical & Experimental Pathology
ISSN 2977-5817 (Online)
Copyright © Asia Pac J Surg Exp & Pathol. This
work is licensed under a Creative Commons AttributionNonCommercial-No Derivatives 4.0 International (CC BY-NC-ND 4.0)
License.